Journal of Creation 36(2):97–105, August 2022
Browse our latest digital issue Subscribe
Interface systems and continuous environmental tracking as a design model for symbiotic relationships
There is need for a design model of symbiotic relationships in young-age creationism. Symbiotic relationships are crucial for the functioning of healthy biospheric processes and ecosystem stability. Philosophical naturalists posit that these relationships evolved, and co-evolved later as natural selection, initially focused on struggle and competition in simple organisms, led to greater complexity and cooperation through system self-organization. Alternatively, God created complex cooperative systems with astounding complexity from the beginning. He initially created organism archetypes programmed for holistic relational interaction, which is an important element in proposed creation-based species concepts. We also interpret extant species interactions in the light of a planet groaning with dysfunction and death. Therefore, we propose a new design model of symbiotic relationships using human-engineered interface systems as viable analogues for understanding and describing them. A mind is the only known origin of interface systems. If God designed interface systems into creatures, then it is reasonable that their harmonious operation would greatly exceed anything man has devised. A model of interface design has great potential for future understanding of organism interactions, biomimetics, systems ecology, Earth stewardship, and, most importantly, recognizing God’s invisible attributes in the physical creation.
A design model of symbiotic relationships is needed within the larger model of young-age creation.1 It should be both testable and robust in its explanations for these relationships. Symbiotic relationships are complex and ubiquitous in nature, crucial for the functioning of Earth processes, community relationships, and a primary focus of systems biology, ecological research, and Earth stewardship.2 ‘Symbiosis’ (Gr: living together) was first termed symbiotism (German: Symbiotismus) by botanist Albert Frank.3 Later, botanist Anton de Berry first coined symbiosis in a speech, as he referred to long-term and intimate alliances between two or more different species.4,5 Though definitions and examples have changed and are changing over time, the general consensus is that these long-term associations can have varying effects within intimate relationships. There is much discussion surrounding the details of these relationships and what may or may not be considered a symbiosis, but they are out of the purview of this paper. The purpose of this report is to briefly review the types of alliances and proposed naturalistic mechanisms for the origins of those alliances.4,6 We also propose a new model for symbioses using human-engineered continuous environmental tracking and interface systems as viable analogues for understanding and describing biological relationships.7-9
An explanation consistent with Scripture is design-based and organism-focused. It expects autonomous entities with innate design-adapted capacities that were initially created to enable each to work together as parts of larger, non-violent, cooperative systems. These systems would yield results (some synergistic) that facilitate populations to fill the earth and involve key biogeochemical processes crucial for the health of the planet. This wondrous design would also reflect the good, relational, beautiful, and awesome character of the Creator behind it. Cooperative relationship would not only be at the multicellular level, but extend all the way up to, for example, fungi working together with the seeds of plants that together enable them both to live in colder climates, use less water, and/or produce higher yields.10 However, it is also important to note that this world groans with pain, dysfunction, and death because of man’s rebellion against God.11 Questions among young-age creationists surrounding what constitutes biblical life and biblical death are debated, and more insight is needed on these topics in order to better characterize the origin of parasitism in a young-age paradigm, where initial relationships were very good.12 Creationists should lead the way in explaining these highly complex interactions as the work of a Creator and intimate designer who wants to be known, desires to rescue us from ourselves, whose love is reflected in highly complex systems designed for life provision and sustainment, and who is deserving of great glory and honour.13
Symbiosis classification categories
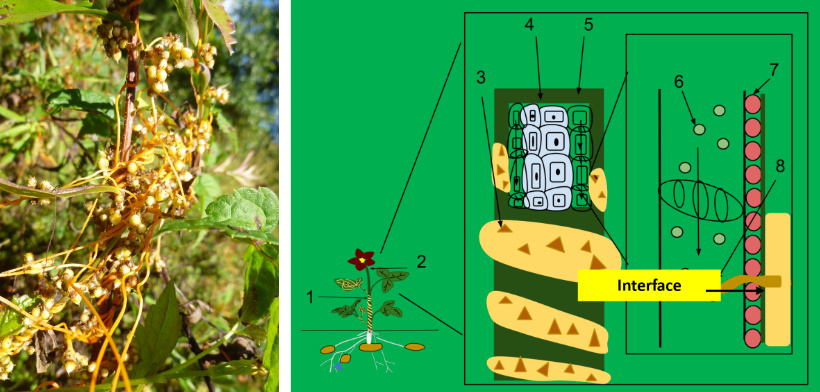
Symbiosis classification can be complex and confusing, as there are several subcategories of relationship characteristics.6 Parasitism, mutualism, and commensalism are classified as functional relationships and describe how symbionts interact with each other. Parasitic relations describe intimate relations of one species taking nutrients from another that does not generally result in death of the host (see figure 1).14,15 Parasites can be contrasted with parasitoids, which are insects with a free-living stage that have intimate relationships with a host, during parts of its lifecycle, but generally kill the host in order to complete its lifecycle.15 It has been estimated that nearly all organisms on the planet are host to at least one parasite species.12 As will be discussed below, some species can become parasitic and/ or pathological under certain circumstances. Commensal alliances have not been well researched. They have been historically defined as relationships where one member is not affected by the relationship, but the other is benefited (see figure 2).16,17 However, some commensal relationships have been reclassified as mutualisms with further research. Some investigators are suggesting that they are more complex than was thought, because interactions are dependent on groups of phenomena depending on ecological processes and relational components.18 Mutualistic symbioses are alliances where all involved benefit (see figure 3).19,20 There are also categories of structural relationships. Francis simplifies the structural categories into two broad groupings: the location of symbionts in relationship to one another and the degree of dependence they have for one another.3 There are three structural subcategories of locations that symbiologists have identified. Endocytobiosis occurs when one symbiont lives inside the cell of another, such as bacteria living inside protists that reside in the gut of termites.21 Exocytobiosis is where one symbiont is external to the other, as in lichens, and endosymbiosis occurs when one symbiont is extracellular but operates in internal spaces, such as protists in the gut lumen of termites.3,21 As for the degree symbionts are dependent on one another, they can either be facultative or obligate. The above overview is a surficial view of the ubiquitous types of symbioses found in nature, but how might these alliances have originated?
An overview of a naturalist understanding of the origin of symbioses
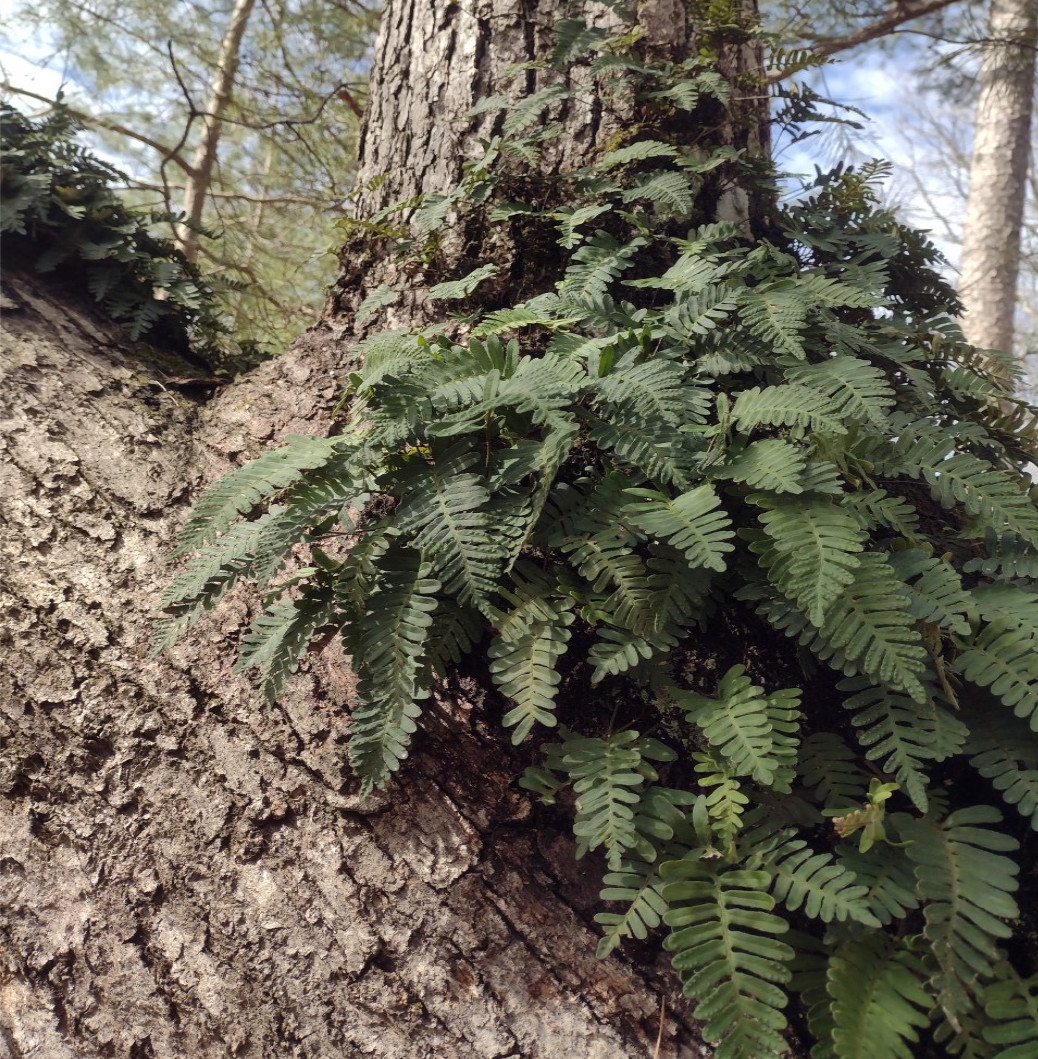
Though some authors have claimed that universal descent with modification is not robust enough to fully explain the origin of symbiosis, evolutionists are in general agreement that natural selection and mutation events can consistently address reasonable explanations for their origins, which are assumed to begin with simple organisms that were not symbiotic.22 Some complex mutual symbioses, such as lichens and arbuscular mycorrhizal fungi, have been conventionally dated 600 million and 400 million years respectively.23,24
Historically, symbiotic alliances were not thought to be common, nor were they considered phylogenetically significant. Since Darwin, much of the evolution emphasis has focused on struggle and competition, but cooperation (e.g. kin selection, group selection, reciprocity, and by-product mutualism) is also crucial in an evolutionary paradigm in order to understand symbiotic associations and the success of mammals.25 A landmark paper by Margulis (Sagan) on endosymbiotic theory was a turning point in a naturalistic understanding of eukaryotic cell evolution.26 Though it took time for the scientific community to acknowledge, and not all of her hypotheses were correct, the evolutionary community is in general agreement that her key explanations for cells merging into one another through cooperation is consistent with an evolutionary understanding for the origin of eukaryotic genomes, chloroplasts, and mitochondria.27 Simple cells continued to evolve in organismal and relational complexity, and symbiotic alliances were considered key evolutionary events. Two general directions of investigation have been the focus of symbiotic origins: 1) how antagonistic and autonomous organisms entered into mutually beneficial alliances, and 2) the capturing of an organism by another organism with a symbiotic history.28
It is important to keep in mind that the degree of association is assumed to be based on the energetic costs and benefits that are inherent for an association to be established.6 In systems ecology, it is all about energy and efficiency. Many would argue that initial organisms were produced by random natural processes, but, as more and more organisms interact in a system, natural selection drives that system into increasing specialization, cooperation, efficiency, and complexity, resulting in ecosystem resilience and stability.29 For example, competitive relationships are not considered energy efficient because some of the precious energy required for life (e.g. foraging, mating, thermoregulation) is used to compete for resources. Therefore, natural selection can drive competitors into more energy efficient interactions such as microhabitat separation (e.g. stream insects inhabiting substrate while others live at the surface), temporal separation (e.g. hawks hunting by day and owls by night), resource partitioning (e.g. Galápagos finch beak diversification allowing for a variety of seed resources for a diversity of finches) and mutualisms (lichenized fungi, and/or algae/cyanobacteria cooperating as one organism).
As evolutionary processes continue through time, symbiotic interactions can hurt or help their partners, depending on the natural circumstances and identified genetic mechanisms. For example, Douglas30 describes a Photorhabdus bacterial species in a mutual alliance with a heterorhabditid nematode residing in its gut. The nematode provides food and shelter to the bacterium and the bacterium helps the worm in its healthy growth and development. But the nematode is an endoparasite of soil insects. When the bacteria are released into the insect by the nematode, the bacteria are pathogenic to the insect. The bacteria multiply and thrive on insect nutrients and produce toxins that inhibit other parasites. The nematode will also feed on the bacteria as the insect weakens. As conditions worsen, the worm produces a non-feeding nematode form, gets colonized by remaining bacteria, and returns to the soil to find another insect. The genetic mechanisms for this complex behaviour are carefully orchestrated and some have been identified. HexA, for example, positively regulates for mutualism and negatively regulates for pathogenesis.31 Other genes have been identified up- and down-regulating for pathogenesis and mutualism as well. There are many relationships like the above, and researchers suggest that these relationships began as antagonistic pathogens and that genetic circuitry evolved to have a balanced control of mutualism and pathogenesis because benefits outweighed costs and enabled increased fitness for future generations.
Douglas32 describes two types of evolutionary scenarios that might explain the origins of symbiotic relationships. They include the transition mechanisms from antagonistic to mutualistic relations and partner capture. These events are complex, and many organism examples could be given to illustrate each. Antagonistic to mutual relationships may have happened by amelioration or addiction. Amelioration may happen when virulence decreases in a way that cooperation produces greater fitness for the parasite. Addiction may happen when one organism becomes dependent on a partner without benefit. Though symbiotic addiction has been observed and described, it does not seem to be prevalent. The other possible event is called partner capture, and that can occur when organisms with a history of symbiosis acquire a new partner, as in the case of mycorrhizal associations. Suffice it to say that biologists have observed countless examples of associations where the above events are plausible, and research is shedding light on the details of these complex associations, even at the genetic level. But what is often missing are the most complex questions of what detailed properties of each autonomous system must be necessary in order for them to interact in intimate and intricate ways.
An alternative design hypothesis for the origin of complex alliances
A philosophical naturalistic premise for the origin of life and biotic interactions includes randomness and abiogenesis/panspermia of the first cell. Systems are then assumed to self-organize (or self-reinforce) and increase in complexity via natural selection. As young-age creationists, we reject that premise in favour of the Genesis account of created kinds.33 This has ramifications for the species concept issue, because species of creatures are the ones interacting in these close-knit relationships. If an original archetype (kind) of creature was created by a designer with intelligence and understanding, especially when it was designed to interact in complex systems, it is easy to see how conclusions drawn from extant systems could greatly differ from the naturalist view. For example, a young-age creation model would predict the opposite progression from mutualistic relationships to relationships of suffering and harm (e.g. parasitism).34-37 Though evolutionary biologists have many different definitions for a species, the definitions are based on the faulty premise of dismissing the Genesis narrative. Therefore, when analyzing and drawing conclusions about ecological symbioses, it is imperative that creation biologists define the species in a way that is consistent with a biblically based/baraminological paradigm. This process has begun, and young-age creationists are in agreement that a biblical view of species, while rejecting species fixity and moving beyond essentialism, is a product of God’s initial prototype.38 These first organisms were designed to interact with others and fit into larger and more complex systems that would reflect God’s invisible qualities of intelligence, unity, diversity, beauty, provision, and sustainment for all His creatures.39-41 These creation-based species concepts suggest that a biblical/baraminological view of species has better explanatory power for phenomena such as ring species, Cenozoic species stasis, allopatric species, geographical heterogeneity, stable morphology in hybrid zones, cryptic/sibling species, and symbiotic relationships than Mayr’s biological species and other evolution-based species concepts.
We want to emphasize that though organisms do have characteristics of autonomous molecular machines, they are connected and thrive on relationships, and relationship is an invisible attribute of God.42 As the above examples demonstrate, some of these interactions are good in that relationships are beneficial to all involved. Some of these relationships produce suffering and death for one or more symbionts and, in some situations, may move from mutually beneficial to antagonistic within the same life cycle. Evolutionary explanations lack the specific detail of the complex requirements that must happen in order for two or more autonomous organisms to enter into relationship. We believe that initially these relationships began with fully formed organisms designed to work together in very good ways, which is consistent with the Creator’s relational nature but contradictory to an evolution model.43 However, man’s rebellion resulted in God cursing the ground, resulting in the negative consequences of suffering, disease, and death.44,45 All of these considerations are key scaffolding in a model of symbiotic relationships within the larger model of young-age creation.
Biblical life and death
Biological functions include systems that produce defining characteristics of living entities such as metabolism, growth, responding and modifying to environmental conditions, and reproduction. However, ‘life’ itself and consciousness seem to have attributes which are currently beyond the reach of scientific methods to explain how they originated. This is consistent with the idea that ‘life’ is not physical (not measurable) and originates in the Author of life who is also Spirit and not measurable.46,47 In the same way, biblical death may be more than cessation of life; it may include cessation of function and purpose for which it was created, which should drive deeper discussion of these issues regarding relationship design and parasitism/predation in an initially very good world.45 Therefore, we focus on the task of making sense of biomolecular, physiological, or anatomical functions which have been proven decipherable. In fact, because these complex systems operate with such expected consistency, there is the growing tendency to explain biological functions using engineering or design principles. If diverse biological systems do consistently operate by principles similar to human-engineered systems, then these observations could naturally flow into the development of a model for symbiotic relationships.
Engineered biological design
Engineering principles are experimentally (or experientially) verified rules that must be incorporated into designs in order to get them to work for an intended purpose, or to achieve design characteristics such as efficiency, beauty, communication with two or more entities, and the exchange of resources.48 If an engineer wanted to maximize the heat transfer between two fluids flowing through adjoining pipes, then the engineering principle would stipulate that fluids would flow in opposite directions by a principle known as countercurrent flow. Conversely, if an engineer observed a high transfer of heat between fluids in a biological setting, then their knowledge of engineering principles would guide them to suspect that a countercurrent flow system would be discovered. This countercurrent flow system has been discovered in many taxa.49-52 Research demonstrates a remarkable correspondence in design, purpose, and function for many organism systems with similar devices produced by human engineers.53 Organisms are made of systems that often exploit the properties of ‘natural laws’ such as gravity, inertia, and momentum. (For a compelling talk that reinterprets ‘natural law’ as God’s intentional and active working throughout the universe, see Wise’s talk at a recent conference dealing with Historical Adam).54
In the biology subdiscipline of biomimicry, human engineers regularly copy these systems and use them for inspiration in design and to improve human technology.55 Some are calling for merging the fields of biology, computer science, and engineering, because terms and concepts from these disciplines are being applied in the biology laboratory.56,57 Engineering-inspired fields such as integrative systems biology, biomedical engineering, and synthetic biology have more in common with engineering approaches than with traditional biological ones.58
Engineered biological design: working hypotheses and tenets
The rationale for developing a design model of symbiotic relationships would hardly suggest a ‘tweaking’ of current biological theory. Starting from an engineering basis would produce a radically different model. Previous creation models for programmed mediated design and rapid post-Flood intrabaraminic diversification may be consistent with the model discussed below.59,60 A design model of symbiotic relationships seeks to answer similar questions that current evolutionary theory tries to explain. These are: 1) How do we account for the apparent design of organisms suggested by their purposeful behaviours and exquisite fit of form and function? 2) Can nature function as an agent sufficient unto itself to produce life’s observed structure and function, or is an intelligent agent outside of nature needed? 3) Is the mechanism for organic change an independent and internally designed dynamic within organisms themselves, or is it the external environment and its randomly variable conditions controlling mechanisms for change? 4) How does a design model of symbiotic relationships explain the suffering and pain that can come and go as organism interactions change from mutual, commensal, and parasitic if God is good?
We hypothesize that:
- Biological functions will be accurately explained by models developed using engineering principles.
- Studying human engineering practices will accurately inform biological research predictions and will direct researchers to precise characterizations of phenomena.
Moving biology into the realm of engineering may seem extreme, but we predict it will be how biology is practised by future generations of biologists. That said, these understandings do not take away from the wonder, beauty, emotional, and spiritual experiences that come from exploring a forest trail, enjoying a beach sunset, contemplating the relational intelligence between mother bear and her cubs, or investigating the biomolecular interactions of tree roots and mycorrhizal hyphae. Wonder, beauty, curiosity, emotional wellness, and enjoying relationships with other beings are also part of God’s invisible qualities and are consistent with a very special world.
Computational biologist Sara-Jane Dunn gave a fascinating talk about her work at the interface between biology and computation.61 The distinction between basic biological research and its engineering applications, and who is doing either, is unclear. Thus, we now find some biologists and engineers focused on basic research and some in both fields concentrating on applications. Today, investigators acknowledge that research is best handled by a multi-disciplinary approach, but the future will likely see more of a uni-disciplinary approach where the fields are merged, and all must learn their craft by studying beyond their major academic disciplines.62
Based on our hypothesis that studying human engineering practices will direct researchers to precise characterizations of phenomena, at least three tenets provide an engineering-based context for interpreting biological observations.
- Intentionality (teleology). Goal-directed activity to specific ends is observed in creatures essentially as a continuum from the molecular level to the whole organism, to the community, to the ecosystem. Rather than contrive explanations to work around this phenomenon, a design model of symbiotic relationships would characterize interpretations of biological systems in terms of their primary purpose and also embrace the search for ‘purpose’ as a useful guide to research agendas. ‘Top-down’ is the goal-directed approach humans use when designing systems. Thus, within a design model of symbiotic relationships, the ‘top-down’ rule for interpreting biological findings is seen as essential to correctly analyze systems.
- Internalistic. All biological operations arise from identifiable control systems innate to the organism. The form and function of living entities are governed by innate systems. A rule for interpretation is that internal programming—not environmental conditions—will be credited for the successful solution to challenging exposures that may lead to differential survival and reproduction in a population.
- Individualistic. Like all engineered entities, organisms are discreet individuals delineated by definite and distinct boundaries that distinguish ‘self’ from ‘non-self’. A design model of symbiotic relationships expects innate engineered controls will regulate organism–environment relationships as well as organism–organism relationships. An organism’s internal programming specifies (and restricts) only certain external conditions to be stimuli. Therefore, when interpreting how ‘self’ relates to ‘non-self’, individual organisms must be seen as discreet elements of broader systems called ‘ecosystems’. An accurate understanding of the ecosystem as a whole is obtained by accounting for the individual role of each kind of organism as a distinct element fitting within an extensive system. This contrasts with the view of many evolutionists, such as Steven Rose of The Open University, that blur the distinction between individuals and the ecosystem. Rose said, “There is no overriding reason why we should consider ‘the organism’ as an individual rather than ‘the group’ or even ‘the ecosystem’.”63
An engineering-based model of symbiotic relationships (MOSR) recognizes that characteristics of ecosystems emerge from the contributions of each individual in relationship with one another. Individuality, therefore, is not obliterated by seeing creatures as being absorbed into an environmental collective. Thus, no matter how closely two (or more) individuals may operate together in what engineers may call a seamless operation, a MOSR recognizes that there really is a seam and focuses research on what is happening at the seam. We predict that each individual organism will have an interface system that tightly controls how they relate to each other. It is the innate control of these relationships by each individual that determines the characteristic of the ecosystem. Therefore, a primary expectation of a MOSR is to discover corresponding system elements between human-designed contrivances and biological mechanisms performing similar functions. Evidence has been described supporting this prediction.7-9
MOSR expects symbiotic relationships to be regulated by biological interfaces
Recognizing organism relationships is far from explaining the mechanisms enabling that relationship to happen. This is a fundamental question at the foundations of biology: how do two autonomous entities with distinct boundaries either work together or not?
Understanding symbiosis based on design analysis starts by looking for an analogous human-designed relationship, the mechanisms for operation of which are already understood, and seeing if there is a true correspondence between its constituent elements and those elements found within symbiotic biological relationships.
How could engineers overcome dissimilarities and get two autonomous entities with distinct boundaries to work together? There must be a bridging mechanism. A logical solution connects them via an interface. Prominent computer interface designers Kim Clark and Brian Petrini underscore the necessity of interfaces for cooperation. They emphasize that to understand an interface is to understand how autonomous entities can form intimate alliances and cooperate with one another by communicating in such a way that information and product exchanges occur in a harmonious fashion.64,65
Distinctive elements characterize interface systems
Designers use in-depth operational knowledge of both unrelated entities to integrate their functions into three indispensable interface elements: authentication, protocols, and a common medium of conditions mutually accessible to both entities. These three elements constitute the minimal components needed to attain basic functioning of an interface. Removal of any one of them causes an interface system to effectively cease functioning.
Authentication is a key mechanism used to recognize ‘self’ from ‘non-self’. Authentication is a highly regulated and extremely selective process that constitutes one element of an interface system. Systems or behaviours within individual organisms will function as an interface that often mandates disclosure of identifying information, validate that information, authenticate identities, and authorize exchanges with only select entities within an ecosystem.
Protocols are rules, processes, or mechanisms established by the interface designer that work between requestor and provider in order to regulate the relationship. Physical attachment often precedes control. If actual physical contact will be an element of control, protocols specify the physical conditions (i.e. for living things protocols specify the trait(s) that enable(s) physical attachment), which facilitate regulation through physical contact. Therefore, it is common that a uniting element fits together material elements at the boundaries of both entities like the Apollo-Soyuz docking station. One basic way for an autonomous entity (A) to produce a desired outcome from another entity (B) is for A to physically attach to B and take control over B.
There is another type of regulation between autonomous creatures that doesn’t involve physical contact. These outcomes are actually the consequential end product of each creature’s internal processes. The regulation starts when one creature detects specific conditions (i.e. stimuli) caused by another autonomous creature. It takes an elaborate design for one entity to present specific external conditions to an environment, which, when those conditions are detected by a second entity, responds by making a product that is useful to either the first entity or to the community as a whole. One example of highly complex interactions with little direct physical contact is earthworm multiple interactions in ecosystems. One study showed that they improve agroecosystem functions by improving and strengthening bacterial and small animal community interactions by indirect impacts that resulted in an improved and sustainable healthy growing environment for rice crops.66
The Common Medium is a physical condition external to two or more entities. Each entity must have at least one of its traits capable of interacting with the condition. For example, when humans speak to each other they use a common medium of air. Vocal cords, which can compress air into waves, are the trait of one person, and ear drums, which can sense compressed air waves, are the trait of the other person. Other common mediums are chemicals, electricity, or light. So, if the common medium is chemical, then each creature must have some trait that can produce and/or sense chemicals. So, we see a common medium is required in order to connect two autonomous systems and is therefore an absolute condition for an interface.67
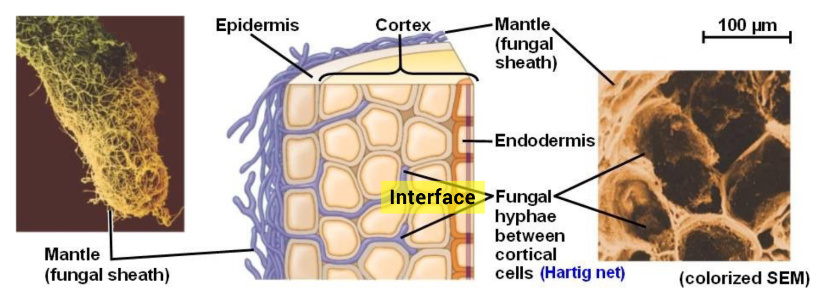
When observing certain organism symbionts, it could look like one species is directly controlling the other, but they are not. We need to remember how engineered interfaces work. Each organism has an interface, but the interfaces are only controlling the organism to which it belongs. This relationship is sometimes tricky to understand. Why? Because in mutualisms, harmonious outcomes are seen, but the interfaces that enable the harmonization aren’t seen. For example, when the fungal network interface of the Hartig net supplies nitrogen and phosphate resources to tree roots and tree roots supply carbohydrates to the fungus, it is easy to assume one might be controlling the other (see figure 3). This is not correct. The fungus and the tree root each control their own resource exchange. Selectionists assert that a long trial-and-error process of coevolution brought about the ability for two completely different taxa to help one another. An engineering-based MOSR would suggest that the immaterial information is controlling these physical operations within the fungus and the tree, which are two distinct, autonomous entities. In this case, if one went searching for where the information that specifies harmonization of the ectomycorrhiza and forest tree roots resides, it would not be found within either but comes from the mind of the Designer.67
In a MOSR, parasitism and commensalism may be the result of the removal/dysfunction/mutation of one or more of the above elements of interface systems. In this view, parasitism is the violation of distinct boundaries of one entity upon another. This is in contrast to mutualism, which does not violate boundaries between entities.
Normally, free living nematodes seem to prefer to feed on one genus of bacteria, Pseudomonas, over other bacteria on which they routinely feed.68 However, a nematode (Caenorhabditis elegans) that lives in soils of temperate regions learned to avoid the pathogenic species Pseudomonas aeruginosa and was able to transmit this learned avoidance for approximately four generations. The nematode can detect double-stranded RNA found in the pathogenic P. aeruginosa and it initiates a response. Investigators discovered that changes in the expression of a gene, daf-7, in a specific neuron called ASI, was a likely cause for the worm’s avoidance behaviour.69 These researchers also found substantial changes in the small RNAs in the germline, including the ones called Piwi-interacting RNA (piRNA). As the name suggests, piRNAs interact with piwi genes, which help to regulate stem cell differentiation. Thus, data suggests that the piRNA pathway is critical for inheritance of the behaviour.
The above example illustrates a complexity that far surpasses naturalistic explanations and is an excellent example for MOSR interpretation.
Conclusions
Based on human-engineered analogues, all relationships between biological entities are likely rooted in a basic interface design principle that enables them to interact with each other. Interface design is challenging. A mind is the only known origin of interface systems. It is an information-intensive task to devise physical or logical mechanisms to harmonize independent, often dissimilar, organisms. If God designed interface systems into creatures, then it is reasonable that their harmonious operation would greatly exceed anything humans have devised. Clark and Petrini underscore the importance of an interface designer’s thorough knowledge of all systems.64-66 The Designer needs to understand all of the requirements of the requester while grasping all aspects of provider capabilities. Thus, for creatures to harmonize, the interface designer must foresee the outcome desired for each creature that will result from the relationship and have in-depth knowledge of their phenomenally complex systems. Given that all creatures seem to be linked into vast ecosystems, this indicates that the Engineer of all of these interfaces possesses an incomprehensible amount of knowledge.
The ecosystem–interface model is powerful confirmation for an engineering-based approach to symbiotic relationships. Evolutionists struggle to explain the origin of information for even an individual organism. But when it comes to highly interfaced relationships such as lichens, plants/pollinators, and mycorrhizal fungi, evolutionary naturalists must claim that these relationships were never elements of any overarching plans. Their solution is an appeal to everything coevolving together. When one reads ‘coevolution’ in evolutionary literature, then that should prompt a biological researcher to look for two or more interfaced organisms. But, if ‘coevolution’ amounts to no more than a declaration, then we must ask, where are the regulatory plans located for a world of innumerable and diverse ecosystems? From a model of symbiotic relationships viewpoint, they exist in the mind of the omniscient ecosystem Engineer, the Lord Jesus Christ.
Acknowledgments
We would like to thank the anonymous reviewers for their helpful and insightful suggestions on earlier manuscripts.
References and notes
- Hennigan, T., Toward a biblical basis for ecology, with applications in mycorrhizal symbioses in orchids, J. Creation 23(1):78–85, 2009. Return to text.
- Hennigan, T., Toward an understanding of arbuscular mycorrhizal symbioses within a creation model of ecology: implications for godly stewardship and sustainable agriculture, ARJ 2:21–27, 2009. Return to text.
- Francis, J.W., Symbiosis, relationship and the origin of species, Genesis Kinds: Creationism and the Origin of Species, Issues in Creation No. 5, Wood, T.C., and Garner, P.A. (Eds.), Wipf and Stock, Eugene, OR, pp. 163–192, 2009, Return to text.
- Douglas, A.E., The Symbiotic Habit, Princeton University Press, Princeton, NJ, pp 3–5, 2010. Return to text.
- Stubbendiek, R.M., Li, H., and Currie, C.R., Convergent evolution of signal-structure interfaces for maintaining symbioses, Current Opinion in Microbiology 50:71–78, 2019. Return to text.
- Paracer, S. and Ahmadjian, V., Symbiosis: An introduction to biological associations, 2nd edn, Oxford University Press Inc., New York, pp. 1–13, 2000. Return to text.
- Guliuzza, R.J. and Gaskill P.B., Continuous environmental tracking: an engineering framework to understand adaptation and diversification; in: Whitmore, J.H. (Ed.), Proceedings of the 8th International Conference on Creationism, Pittsburg, PA, pp.158–184, 2018. Return to text.
- Hennigan, T. and Guliuzza, R.J., The continuous environmental tracking hypothesis—application in seed dormancy and germination in forest ecosystems, J. Creation 33(2):77–83, 2019. Return to text.
- Guliuzza, R.J. and Sherwin, F., Design analysis suggests that our ‘immune’ system is better understood as a microbe interface system, CRSQ 53(2):123–139, 2016. Return to text.
- Jones, N., Food fuelled with fungi, Nature 504:199, 2013. Return to text.
- Genesis 3; Romans 8. Return to text.
- Blaschke, J., Toward a young earth model of parasite evolution, J. Creation Theology and Science Series B: Life Sciences 8(1):1–5, 2018. Return to text.
- Genesis 1 and 2; Psalm 19:1; John 1; Romans 1:20. Return to text.
- Labandeira, C.C. and Li, L., The History of insect parasitism and the mid- Mesozoic parasitoid revolution; in: De Baets K., Huntley J.W. (Eds.), The Evolution and Fossil Record of Parasitism, Topics in Geobiology, vol. 49, Springer, Cham, Denmark, pp. 377–533, 2021 ǀ doi.org/10.1007/978-3-030-42484-8_11, accessed 1 Mar 2022. Return to text.
- Spira, T.P., Wildflowers and Plant Communities of the Southern Appalachian Mountains and Piedmont: A naturalist’s guide to the Carolinas, Virginia, Tennessee, and Georgia, University of North Carolina Press, Chapel Hill, pp. 356–357, 2011. Return to text.
- Gramling, J.M., Epiphytic plants in South Carolina, South Carolina Native Plant Society, Summer 2010, Pages-from-epiphytic-plants.pdf (coastalconservationleague.org), accessed 19 Feb 2022. Return to text.
- Pratts, K.A. and Broderson, C.R., Desiccation and rehydration dynamics in the epiphytic resurrection fern Pleopeltis polypodioides, Plant Physiology 187(3):1501–1518, 2021. Return to text.
- Mathis, K.A. and Bronstein, J.L., Our current understanding of commensalism, Annual Review of Ecology, Evolution and Systematics 51:167–189, 2020. Return to text.
- Stuart, E., Plett, K., Digging deeper: in search of the mechanisms of carbon and nitrogen exchange in ectomycorrhizal symbiosis, Frontier Plant Science 10:1658, 2020. Return to text.
- Nehls, U., Mastering ectomycorrhizal symbiosis: the impact of carbohydrates, J. Experimental Botany 59(5):1097–1108, 2008. Return to text.
- Waidele, L. Korb, J., Voolstra, C.L., Dedeine, F., and Staubach, F., Ecological specificity of the metagenome in a set of lower termite species supports contribution of the microbiome to adaptation of the host, Animal Microbiome 1:13, 2019. Return to text.
- Douglas, ref. 4, p. 2. Return to text.
- Yuan, X., Xiao, S., and Taylor, T.N., Lichen-like symbiosis 600 million years ago, Science 308:1017–1020, 2005. Return to text.
- Taylor, T.N., Remy, W., Hass, H., and Kerp, H., Fossil arbuscular mycorrhizae from early Devonian, Mycologia 87:560–573, 1995. Return to text.
- Paracer and Ahmadjian, ref. 6, p. 6. Return to text.
- Sagan, L., On the origins of mitosing cells, J. Theor. Biol. 14:225–274, 1967. Return to text.
- Lazcano, A. and Peretó, J., On the origins of mitosing cells: A historical appraisal of Lynn Margulis endosymbiotic theory, J. Theor. Biol. 434:80–87, 2017. Return to text.
- Douglas, ref. 4, p. 24. Return to text.
- Perry, D.A., Self-organizing systems across scales, Trends in Ecology and Evolution 10(6):241–244, 1995. Return to text.
- Douglas, ref. 4, p. 25. Return to text.
- Douglas, ref. 4, p. 27. Return to text.
- Douglas, ref. 4, pp 29–35. Return to text.
- Wood, T.C, Wise, K.P., Sanders, R., and Doran, N., A revised baramin concept, Occas. Papers of the BSG 3:1–14, 2003. Return to text.
- Sherwin, F., A possible function of Entamoeba histolytica in the creation model, ARJ 2:117–121, 2009. Return to text.
- Loucks, I., Fungi from the biblical perspective, ARJ 2:123–131, 2009. Return to text.
- Purdom, G., The role of genomic islands, mutation, and displacement in the origin of bacterial pathogenicity, ARJ 2:133–150, 2009. Return to text.
- Ingle, M., Parasitology and creation, ARJ 8:65–75, 2015. Return to text.
- Wood, T.C., Species concepts and creationism: beyond essentialism, J. Creation Theology and Science B: Life Sciences 11:8–9, 2021. Return to text.
- Wise, K.P., Species as brushstrokes: the revelatory species in creation, J. Creation Theology and Science B: Life Sciences 11:27–41, 2021. Return to text.
- Wilson, G., Genomic equivalence and speciation: proposing a new criterion for baraminology J. Creation Theology and Science B: Life Sciences 11:42–46, 2021. Return to text.
- Sanders, R.W., Minimal discontinuity species concept: A practicing taxonomist’s attempt to understand species in the context of baraminology, J. Creation Theology and Science B: Life Sciences 11:10–26, 2021. Return to text.
- Romans 1:20. Return to text.
- Genesis 1:26; John 1; Romans 1:20. Return to text.
- Genesis 3; Romans 1–5. Return to text.
- Wise, K.P., Biblical death as a cessation of function, J. Creation Theology and Science Series B Life Sciences 9:1–7, 2019. Return to text.
- Genesis 2:7; John 4:24. Return to text.
- Wise, K.P., Devotional Biology: Learning to worship the creator of organisms, Truett McConnell Publications, Cleveland, GA, pp 33–52, 2016. Return to text.
- Lucas, K., Johnson, N., Beaulieu, W. et al., Bending rules for animal propulsion, Nat. Commun. 5:3293, 2014. Return to text.
- Guliuzza, R., Reverse engineering reveals ideal propulsion design, icr.org/article/ reverse-engineering-reveals-ideal-propulsion, accessed 2 Dec 2021. Return to text.
- Albano, G., Slowskei, L., Puckett, L., and Reynolds, A. Modeling countercurrent arteriovenous heat exchange and blood flow in a finger exposed to cold, eCommons Open Scholarship at Cornell, 2018, ecommons.cornell.edu/handle/1813/57233, accessed 2 Dec 2021. Return to text.
- Aoki, S. K., Lillacci, G., Gupta, A. et al., A universal biomolecular integral feedback controller for robust perfect adaptation, Nature 570:533–537, 2019. Return to text.
- Khammash, M., Reverse engineering: the architecture of biological networks, BioTechniques 44(3):327, 2008. Return to text.
- Kunkel, J., Luo, X., and Capaldi, A.P., Integrated TORC1 and PKA signaling control the temporal activation of glucose-induced gene expression in yeast, Nat. Commun. 10:3558, 2019. Return to text.
- Wise, K.P., facebook.com/truettmcconnell/videos/159297312621535, accessed 2 December 2021. Return to text.
- Socha, J., Snakes that fly—really, youtube.com/watch?v=fVa397fMEv4, accessed 2 Dec 2021. Return to text.
- Savage, N., Computer logic meets cell biology: how cell science is getting an upgrade, Nature 564:S1–S3, 2018. Return to text.
- Pande, V., How to Engineer Biology, blogs.scientificamerican.com/observations/ how-to-engineer-biology/?utm_source=newsletter&utm_medium=email&utm_ campaign=weekly-review&utm_content=link&utm_term=2018-11-14_more-stories, accessed 2 Dec 2021. Return to text.
- royalsociety.org/topics-policy/projects/synthetic-biology, accessed 2 Dec 2021. Return to text.
- Wood, T.C., Mediated design, ICR Acts and Facts Impact Article #363, 2003; icr.org/i/pdf/imp/imp-363.pdf, accessed 2 Dec 2021. Return to text.
- Wood, T.C., Rapid post-Flood intrabaraminic diversification caused by altruistic genetic elements (Ages), Origins 54:1–34, 2002, grisda.org/origins-54005, accessed 2 Dec 2021. Return to text.
- ted.com/talks/sara_jane_dunn_the_next_software_revolution_programming_ biological_cells, accessed on 2 Dec 2021. Return to text.
- Savage, N., Computer logic meets cell biology: how cell science is getting an upgrade, Nature 564:S1–S3, 2018. Return to text.
- Rose, S., The biology of the future and the future of biology; in: Cornwell., J. (Ed.), Explanations: Styles of explanation in science, Oxford University Press, Oxford, p. 138, 2004. Return to text.
- Clark, K.J. and Petrini, B.M., Capturing and analyzing interface characteristics, part 1: capturing integration complexity for BPM and SOA solutions, IBM Developer Works, 7 Dec 2011. Return to text.
- Clark, K.J. and Petrini, B.M., Capturing and analyzing interface characteristics, part 2: reference guide to integration characteristics, IBM Developer Works, 25 Jan 2012. Return to text.
- Liu, T., Chen, X., Gong, X., Griffiths, B.S., Hu, F., and Liu, M., Earthworms coordinate soil biota to improve multiple ecosystem functions, Current Biology 29(20):3420–3429e1-e5, 2019. Return to text.
- Baumgardner, J.R. and Lyon, J.D., A linguistic argument for God’s existence, JETS 58(4):771–786, 2015, Microsoft Word -jets58d.doc (etsjets.org), accessed 2 Dec 2021. Return to text.
- Moore, R.S., Kaletsky, R., and Murphy, C.T., Piwi/PRG-1 argonaute and TGF-β mediate transgenerational learned pathogenic avoidance, Cell 177(7):1827–1841, 2019. Return to text.
- Kaletsky, R., Moore, R.S., Parsons, L.L., and Murphy, C.T., Cross-kingdom recognition of bacterial small RNAs induces transgenerational pathogenic avoidance. bioRxiv preprint. www.biorxiv.org/content/10.1101/697888v1.full. pdf, 2019, accessed 2 Dec 2021. Return to text.
Readers’ comments
Comments are automatically closed 14 days after publication.